The energy efficiency of a system or device that converts energy is measured by the ratio of the amount of useful energy put out by the system (“output energy”) to the total amount of energy that is put in (“input energy”) or by useful output energy as a percentage of the total input energy. In the case of fuel cells, useful output energy is measured in electrical energy produced by the system. Input energy is the energy stored in the fuel. According to the U.S. Department of Energy, fuel cells are generally between 40–60% energy efficient. This is higher than some other systems for energy generation.
The theoretical maximum efficiency of any type of power generation system is never reached in practice, and it does not consider other steps in power generation, such as production, transportation and storage of fuel and conversion of the electricity into mechanical power. However, this calculation allows the comparison of different types of power generation. The maximum theoretical energy efficiency of a fuel cell is 83%, operating at low power density and using pure hydrogen and oxygen as reactants (assuming no heat recapture) According to the World Energy Council, this compares with a maximum theoretical efficiency of 58% for internal combustion engines. While these efficiencies are not approached in most real world applications, high-temperature fuel cells (SOFC and MCFC) can theoretically be combined with gas turbines to allow stationary fuel cells to come closer to the theoretical limit.
The tank-to-wheel efficiency of a fuel-cell vehicle is greater than 45% at low loads and shows average values of about 36% when a driving cycle like the NEDC (New European Driving Cycle) is used as test procedure. The comparable NEDC value for a Diesel vehicle is 22%. In 2008 Honda released a demonstration fuel cell electric vehicle (the Honda FCX Clarity) with fuel stack claiming a 60% tank-to-wheel efficiency.
The efficiency of the fuel cell stack (EffFC), the utilization of the hydrogen (UtilH2) and power consumed by the balance of plant components (PowerBOC) determine the total system efficiency:
Effel, sys = EffFC * UtilH2 * (1-(Power BOC / Power Fuel cell system ))
Efficiency gains can be obtained by
- operating at a high cell voltage; MEAs that offer high power densities at high cell voltages are clearly to be preferred: The fuel cell efficiency for hydrogen/oxygen fuel cells, can be obtained by dividing the cell voltage at operation by 1.23 V. Hydrogen/oxygen fuel cells operated at 0.7 V thus have an electrical efficiency of 0.57. This energy efficiency number is based on the lower heating value of hydrogen.
- maximizing the utilization of hydrogen. Open systems with low hydrogen stoichiometries, or closed systems with low purging frequencies, lead to less waste of hydrogen and thus to an increased efficiency.
- minimizing the flow of air. As the flow of air is generally a factor of 4 higher than the flow of hydrogen, the energy needed for supplying this air can pose a significant parasitic loss.
- minimizing the pressure drop at anode and cathode. The pressure drop of the flow field and manifolds requires an increase of the reactant pressure at the inlet that directly leads to an increase in parasitic energy consumption.
Gibbs Helmholtz Equation
The Gibbs–Helmholtz equation is a thermodynamic equation used for calculating changes in the Gibbs energy of a system as a function of temperature. It is named after Josiah Willard Gibbs and Hermann von Helmholtz.
The equation is:
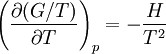
where
H is the enthalpy,
T the absolute temperature and
G the Gibbs free energy of the system, all at constant pressure p
The equation states that the change in the G/T ratio at constant pressure as a result of an infinitesimally small change in temperature is a factor H/T2.