A thermal field, or geothermal field, is the space taken by the installation of a geothermal system.
In a geothermal system, wells are dug in the ground at regular interval. It is the space occupied by these wells that is called a thermal field or a geothermal field. The thermal field, or geothermal field, may consist of a variable number of wells, depending on the intensity of energy sought.
It is an area of the Earth characterized by a relatively high heat flow. The anomolously high rate of heat flow may be due to present, or fairly recent, orogenic or magmatic activity, or to the radioactive decay of isotopes of K, Th, and U where these occur at very high concentrations in crustal granites (hot dry rocks). In sedimentary basins, low thermal-conductivity values for the rocks are balanced by high thermal gradients, thus maintaining a constant heat flow. The high thermal gradients raise the temperature of deep, permeating water. Extraction of the water up deep boreholes provides surface water at temperatures useful for space heating. Hot springs and fumaroles can be important surface manifestations of a geothermal field.
A thermal field, or geothermal field, is the space occupied by the installation of a geothermal system. In a geothermal system, wells are dug in the ground at regular interval. It is the space occupied by these wells that is called a thermal field or a geothermal field. The thermal field, or geothermal field, may consist of a variable number of wells, depending on the intensity of energy sought.
In an geothermal system the processes can be described schematically as ‘convecting water in the upper crust of the Earth, which, in a confined space, transfers heat from a heat source to a heat sink, usually the free surface’ (Hochstein, 1990). A geothermal system is made up of three main elements: a heat source, a reservoir and a fluid, which is the carrier that transfers the heat. The heat source can be either a very high temperature (> 600 °C) magmatic intrusion that has reached relatively shallow depths (5-10 km) or, as in certain low-temperature systems, the Earth’s normal temperature, which, as we explained earlier, increases with depth. The reservoir is a volume of hot permeable rocks from which the circulating fluids extract heat. The reservoir is generally overlain by a cover of impermeable rocks and connected to a surficial recharge area through which the meteoric waters can replace or partly replace the fluids that escape from the reservoir through springs or are extracted by boreholes. The geothermal fluid is water, in the majority of cases meteoric water, in the liquid or vapour phase, depending on its temperature and pressure. This water often carries with it chemicals and gases such as CO2, H2S, etc. Figure below is a greatly simplified representation of an ideal geothermal system.
The mechanism underlying geothermal systems is by and large governed by fluid convection. Convection occurs because of the heating and consequent thermal expansion of fluids in a gravity field; heat, which is supplied at the base of the circulation system, is the energy that drives the system. Heated fluid of lower density tends to rise and to be replaced by colder fluid of high density, coming from the margins of the system. Convection, by its nature, tends to increase temperatures in the upper part of a system as temperatures in the lower part decrease
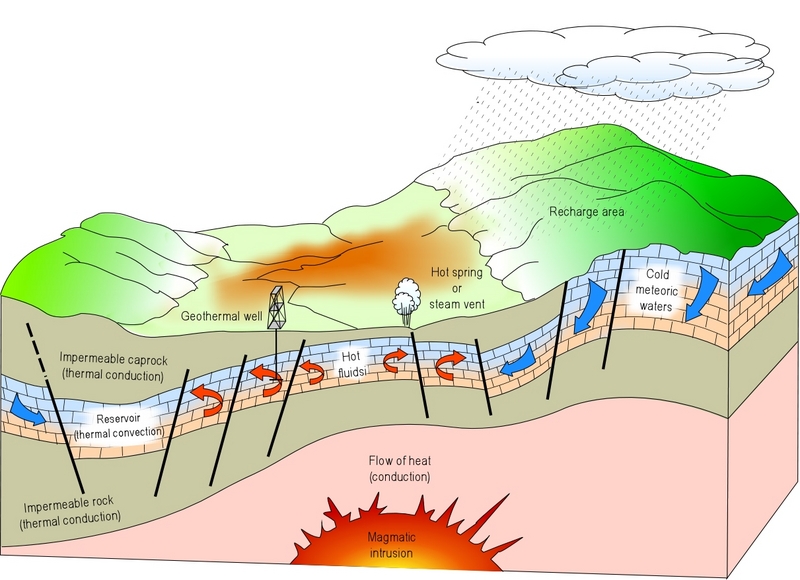
Of all the elements of a geothermal system, the heat source is the only one that need be natural. Providing conditions are favourable, the other two elements could be ‘artificial’. For example, the geothermal fluids extracted from the reservoir to drive the turbine in a geothermal power-plant could, after their utilization, be injected back into the reservoir through specific injection wells. In this way the natural recharge of the reservoir is integrated by an artificial recharge. For many years now re-injection has also been adopted in various parts of the world as a means of drastically reducing the impact on the environment of geothermal plant operations.
Artificial recharge through injection wells can also help to replenish and maintain ‘old’ or ‘exhausted’ geothermal fields. For example, in The Geysers field in California, USA, one of the biggest geothermal fields in the world, production began to decline dramatically at the end of the 1980s because of a lack of fluids. The first project of this type, the Southeast Geysers Effluent Recycling Project, was launched in 1997, to transport treated wastewater for 48 km to the geothermal field. This project has led to the reactivation of a number of power plants that had been abandoned because of a lack of fluids. In the second system, the Santa Rosa Geysers Recharge Project, 41.5 million litres per day of tertiary treated waste-water will be pumped from the Santa Rosa regional sewage treatment plant and other cities through a 66-km pipeline to The Geysers field, where it will recharge the reservoir through specially drilled boreholes.
In the so-called Hot Dry Rock (HDR) projects, which were experimented for the first time at Los Alamos, New Mexico, USA, in 1970, both the fluid and the reservoir are artificial. High-pressure water is pumped through a specially drilled well into a deep body of hot, compact rock, causing its hydraulic fracturing. The water permeates these artificial fractures, extracting heat from the surrounding rock, which acts as a natural reservoir. This ‘reservoir’ is later penetrated by a second well, which is used to extract the heated water. The system therefore consists of (i) the borehole used for hydraulic fracturing, through which cold water is injected into (ii) the artificial reservoir, and (iii) the borehole used to extract the hot water. The entire system, complete with surface utilization plant, could form a closed loop (Garnish, 1987).